Today I’m trying something a bit more challenging than before. I’m going to talk about chemical reactions. For over a year I’ve talked about easy things, like string theory, dark matter and dark energy, but today I’ll dive into the deep end with reactions.
You see, my quest for the theory of everything began with a series of thought experiments. What I needed to understand was how lignin reacts with formaldehyde, because this is one of the most common types of chemistry that is done with lignin. Except usually the reaction is lignin reacting with phenol and then a reaction with formaldehyde. This reaction gives as a commercial plywood adhesive, such as UPM Wisa BioBond.
Except I didn’t want to involve phenol in the reaction. I just wanted lignin to react with formaldehyde. The problem with this reaction is that lignin is a heterogeneous polymer, or a polymer that cannot be described with a simple chemical figure. Indeed, this is what lignin looks like according to Wikipedia:

The problem with the above image was that I couldn’t really use it for simplified images. So, I ended up illustrating lignin as a chain with different sorts of boxes. Some boxes were illustrated with one color to show that they could react with formaldehyde and some boxes with a color illustrating that they were unreactive.
Exactly why I did this is quite complicated but suffice it to say that I needed to make some kind of an illustration of lignin, because a scientist needs tools for visualization.
If I recall correctly, at some point, I illustrated lignin with different colored spheres. Assume a spherical cow, as they tend to say in physics. Almost surprisingly, while this approach was ultimately quite false, in the end, it led me to the right solution. As I recanted this story a year ago, you can read the post if you’re interested.
In the post I talked about the most common bond in lignin, the β-O-4 bond. I already explained how the packing of monolignols leads to the double bond of one monolignol to be right beside a phenol of its neighbor, leading the double bond to open and accept a hydrogen of the phenol.
Here is an illustration of the principle, that I just realized accidentally adds an additional oxygen to the structure. Luckily, I haven’t tried to publish this.
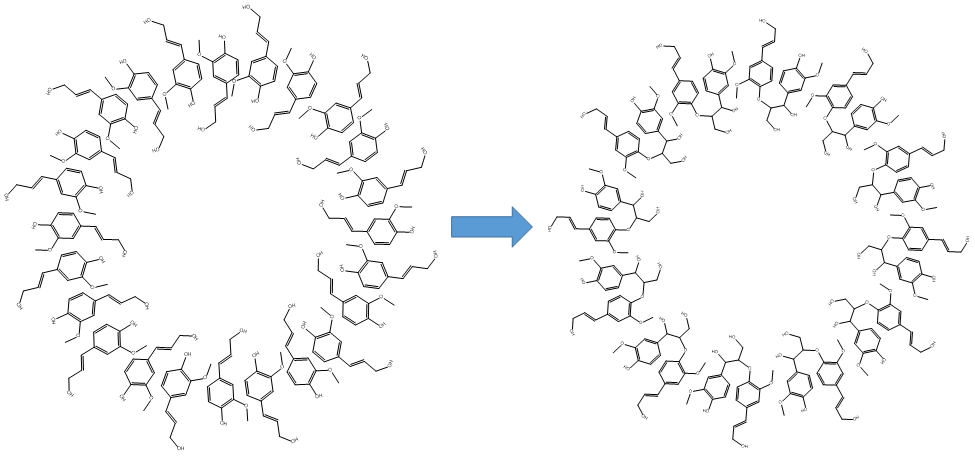
If you zoom in, you can see the hydrogen bond better:
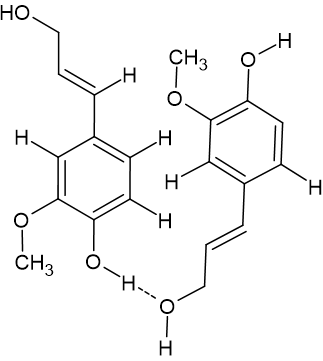
It’s the dashed line. From this 2D representation it’s hard to imagine how the oxygen (O) connected to the hydrogen (H) with a solid line can somehow jump to the carbon (only shown as a line, because we chemists are too lazy to write C) that includes the double bond. The simple answer is that us chemists always ‘cheat’ when we draw 2D structures. If there is no way to show the true 3D structure of a molecule in this illustrative way, we just use the London Tube map model, and ignore true distances to be able to convey the main concept clearly. In reality the 3D alignment of the two monolignol molecules has to be such that there probably should be an intermediate stage with both a hydrogen bond and this new bond. Perhaps a bit like this:
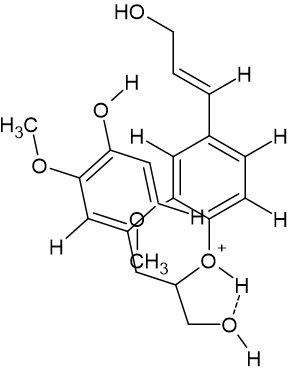
But don’t assume this is that much more accurate than the image before. The important thing to understand is that the hydrogen bond most probably doesn’t open until a covalent bond has formed that is ‘stronger’ than it is. Exactly what takes place is a bit fuzzy to me. Most probably anything published on the topic that is backed by mathematics and experimental evidence is correct, but there is always a danger that there is a ‘competing story’ that is at least a bit off.
So, now we’ve established that there are these very specific β-O-4 bonds at the inside of the monolignol nanotubule. Why wouldn’t there be these same bonds at the outside? It gets a bit complicated, but my intuition says that the same squeezing action that pushes the reactive parts next to the hydrogen bond to react at that very specific spot, causes a stretching action on the outer surface of the monolignol nanotubule, which puts completely different reactive groups next to each other.
Already quite a long time ago, I can’t even remember exactly when, I realized that I can illustrate these different bond types by aligning helices of monolignols (or more specifically lignin dimers) of neighboring toroidosomes and get the exact bonds found in literature. Like this:
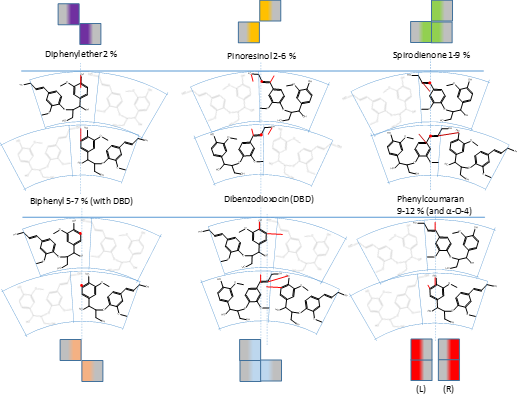
I think for the moment it’s impossible for me to show this in a way that’s understandable for a non-chemist. Possibly it isn’t even clear enough for a chemist yet. I’ll need to work on this to make it intelligible to others.
And to confuse you even more, the lignin polymer doesn’t have just one chemical bond besides the β-O-4 bond. In fact, the lignin polymer is relatively long. From the top of my head I recall it being on average over twenty monolignols long. Because of this, I also made a chart of the which bonds can exist next to which. The initial table looks like this:
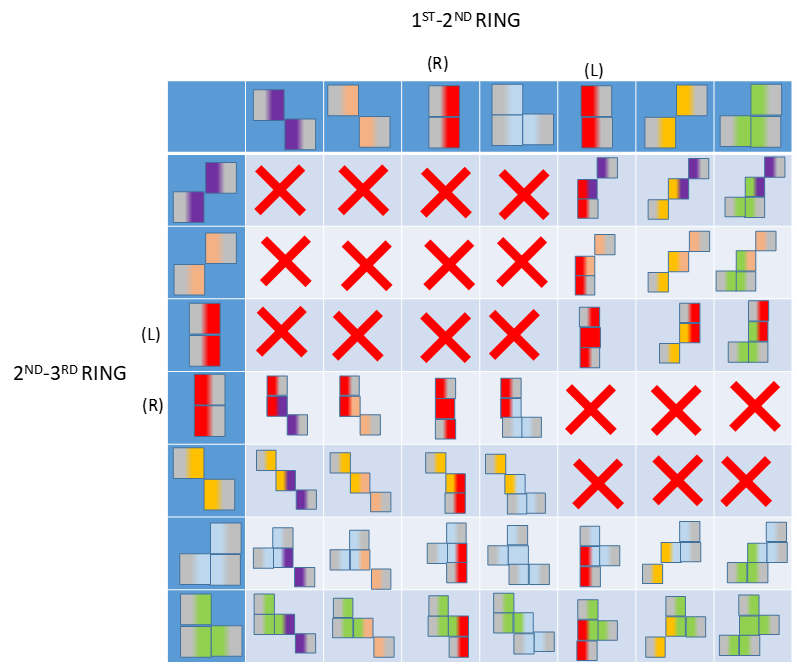
I know it probably doesn’t say much, but it’s quite an important starting point to understand the complex suprapolymeric structure of lignin. Suprapolymeric meaning several polymers being connected together.
And the important thing to understand here is that nothing else changes place during this whole time, apart from the minor movement of the functional groups. Otherwise, the nanotubular shape of the monolignol remains the same.
Yeah, this is pretty complex, even for a lignin chemist. But this is the first practical application of the Theory of Everything. So, if any of the predictions I make here are verified experimentally, it’s also a big feather onto the cap of the Theory of Everything. Hopefully I’ll learn to explain these concepts with simpler terms in the near future.
Comments