The Structure of (Plant) Life
- Kalle Lintinen
- Aug 18, 2022
- 11 min read
While I have written very all-encompassing posts, what I'm about to write now, relates the most to our LignoSphere product. I mentioned in my previous post that in the May of 2020 I had the epiphany that our biological raw material lignin had to have a tubuluar primary structure.
Let's rewind a bit. I started working on lignin spheres in 2014, the same year a Chinese group had published their existence. Lignin is a major side-product in the production of pulp, which is used for paper and cardboard. As a matter of fact, there is almost as much lignin in wood as there is cellulose, the building block of pulp.
There are a couple of thing you need to understand first about lignin. First of all, there is still an understanding that unlike all biomaterial, lignin has no primary structure. There are two reasons for this belief. The primary one is that no structure has been found. Well, this is not exactly right. It has been known for over ten years that lignin is found in a structure called hemicellulose-lignin modules (HLM). But it was not considered HLM could somehow be the primary structure of lignin. The secondary reason for this belief is that unlike most other biopolymers, it's structure seems to be all over the place.
You see, lignin is a polymer, whose buildding blocks, monolignols, are biosynthesized within the plant, in its cytoplasm. But lignin itself is not formed inside the plant cell, but outside of it, building its secondary cell wall. The idea still among most people working in the field is that the plant has a micromanagement mechanism that guides the monolignols between cellulose fibers. What people are looking for is what the components for such a mechanism are.
And then comes the gory part. To extract cellulose from plants (mostly wood), you need to bludgeon your wood into pulp (pun intended). The most common way is the kraft process. In it, you first cut wood into chips and in hot mixture of water, sodium hydroxide (NaOH), and sodium sulfide (Na2S), the chips are broken down into its component parts of cellulose, lignin and hemicellulose. Except that the process does serious harm to lignin and hemicellulose. So much so, that lignin becomes a black gunk called black liquor.
But don't get me wrong. Gone are the days when the gunk would have been spilled to the waterways to make the environment sick. Even the sulfuric compounds from the process that were a major contributor to acid rain from my childhood arent't much a problem anymore. But back in the day, things were different. Pulping was a huge environmental hazard and still even in my childhood, a trip to the neighboring city of Tampere passed such a mill, with the stench of rotten egg from the plant wafting several kilometers to the highway to make that leg of the journey very unpleasant.
Nowadays lignin is put into use. Indeed a significant fraction of the energy produced in Finland comes from lignin. More specifically lignin and other wood fuel (with high lignin content) account for over half of the renewable energy produced in Finland. And while its use as a fuel should not be frowned upon, it has much greater potential than that. For instance Stora Enso has built a huge facility in Sunila Kotka to purify lignin for value-added applications, such as batteries.
The use of lignin in such a way requires quite a step from the nasty black liquor to battery-ready material. To some extent it started with a start-up called Innventia, who invented the LignoBoost purification process. The technology was purchased by Valmet, who in turn built the purification plant to Stora Enso. Now there's several tens of thousands of tonnes of purified lignin available. And not just in one plant, but in several plants all over the world.
So this is where I came in. There were several people working at Aalto University on the lignin spheres, me included. While I was working on my thing, a master's student called Miikka Lievonen was working on the basic process of converting an organic solution of lignin to spheres by inserting the solution into water. The original paper had required chemical modification for the lignin, but Miikka was getting ok results without the modification, but it wasn't prefect. We had the idea that if he would add the lignin solution into a dialysis tube and the tube into water, the organic solvent would go gradually out and water go gradually in, making the process more controlled. The idea worked and Miikka got a very nice publication out of it. This got our work rolling.
Cutting the boring bits out, I finally had time to think about lignin in more depth during the Covid lockdown in the spring of 2020. I was quite confident that the ordered self-assembly of lignin into spheres meant that lignin had to have a primary structure, even if it didn't follow a similar easy structure as most other biopolymers. The lockdown forced into a creative process famously used by the painter Salvador Dali. He used a method he called the ‘slumber with a key’, in which he took very short naps and allowed the images concocted by his subconscious to inspire his art. While for Dali, this produced melting clocks, for me, this resulted in tubes.
For a long time, I had tried to use a rough model of lignin that wouldn’t need to be ‘right’ in the traditional sense of the word, but ‘right enough’ so that I could model how it would react and behave in the process of self-assembly. This is what one of my physics professors called “assume a spherical cow”. The concept of a spherical cow in vacuum is an intentional joke to show how wrong you have to start in physics, when building a new model. There are no alternatives. You must start from a blank slate and only add as much complexity as is required for the problem at hand. Sometimes this means that the starting premise is wrong, but usually this allows one to see whether the studied system follows any mathematical principles.
The rough model that I used was not a spherical cow, but it was spherical lignin. In the model I used colored spheres to illustrate different regions of the polymeric lignin. The polymer was a linear chain of grey and blue spheres. Grey denoted the regions that were unreactive, and blue denoted the regions that were reactive. Once reacted, the color was switched to yellow. Next, I illustrated the self-assembled spheres as a lattice of tightly packed spheres, where I could illustrate how the reacted regions would be randomly interspersed with the unreacted regions, in a sea of unreactive regions. While I knew this model wasn’t exactly right, it had served me quite well in conceptualizing the problem of two neighboring lignin molecules reacting with each other.
Now that I couldn’t be in the lab doing actual experiments, I decided to do thought experiments and revisit the spherical cow model. I already had a relatively good hunch that lignin wasn’t present as dense spheres, but to change the model from a sphere, there would have to be a counter hypothesis, an alternative model. It was somewhat obvious that the outside of lignin pointing towards water should be somewhat attracted to water, or hydrophilic, as they say in chemistry. It also seemed that part of the complex molecules was more hydrophilic, and part was hydrophobic, or repelled water. One way to overcome this apparent contradiction would be if the molecule was twisted into a tube shape, with the hydrophobic parts would be facing each other and the hydrophilic parts would be split in half, with both the outside and the inside being hydrophilic. When this though entered my head, I realized that this shape explained a lot of small observations. I had already read somewhere that when the smallest observed nanoparticles of lignin form, they have water at their core. I also knew that the self-assembly of the lignin spheres to properly take place, there should be less than three weight percent of lignin present in the mixture. While I had previously pondered what the reason for this could be, I had left it at that. However, if the self-assembly involves hollow lignin tubes filled with water, it would be just natural that the water content should be high when self-assembly takes place.
Then there was a the β-O-4 problem. You see, most chemical reactions in nature are 100 % perfect. That is, organisms have enzymes that causes just one type of reaction to take place, which means that they do not have to worry about unwanted side reactions. Lignin, however, reacts in a completely different manner. Plant cells produce the already mentioned starting compounds, monolignols, that undergo polymerization reactions when young plants become woody. The really odd thing is that there are roughly ten different possible reactions. Of these several reactions, the β-O-4 reaction is by far the most common, comprising roughly half of all of them. To simplify a lot, the monolignol molecule has different segments that all can be involved in the reaction, but the most important part of all is a double bond that opens. While lignin couldn’t be farther from the most common man-made polymer, polyethylene, this part is common. Polyethylene forms when ethylene, a really simple molecule with two carbons bound to each other with a double bond, opens one bond and creates a bond with surrounding ethylene molecules. This can be envisioned as two people holding both of their hands and then releasing the grip of one hand and taking the hand of a person who had previously been holding both hands of another person, but also decided to release their grip. Both in the case of ethylene or people this formed chain can be incredibly long. What happens in monolignols is that one part of the molecule resembles ethylene a bit, with the opening of the double bond. However, the other part that takes the outstretched hand, doesn’t look at all like ethylene. The other side of the monolignol is a phenol. Phenol is a curious creature and extremely common in all living organisms. First, phenol has an aromatic ring. This is where six carbons hold hands to form a ring. But here we have to reveal that carbon really doesn’t have just two hands as in the example above, but four hands. However, these extra hands are usually not available to grip anyone, because they are holding shopping bags, or more specifically hydrogen atoms. But they don’t have to hold the shopping bags. They can lay one or both shopping bags down and hold three or four hands of carbon. What happens in an aromatic ring, is that each of the carbons in the ring have their fourth arms inside the ring, the arm swapping grip with two of the carbons on either side. While this grip-swapping would seem to make the bond looser, the aromatic ring is much stronger than almost any other bond in organic molecules. With phenol, one of the shopping bags held by the six carbons is replaced by an oxygen atom. But this oxygen atom has two hands, so now it holds the shopping bag of hydrogen. So, six carbons in a ring, one oxygen and six shopping bags of hydrogen. The β-O-4 bond is where the double bond of the monolignol opens and one of the carbons takes the hand of the oxygen of the phenol and the other carbon takes the hydrogen shopping bag. But there’s a catch. It’s always the same carbon who takes the hand of the oxygen. The carbon of the double bond closer to phenol is called the α carbon, and in this reaction, he gets the shopping bag. The β carbon is farther away from the phenol and he takes the hand of the oxygen.
Okay, so now you have a rough idea what I’m talking about. Next the million-dollar question, why β-O-4? Nothing that I have thus far said explains this. We can think of the monolignols are broad-shouldered body builders sleeping on an infinitely large mattress with one bodybuilder squeezed right next to another. If all of the bodybuilders’ heads were facing the same direction, their broad shoulders would cause them to pack into a circular shape. This reminds me of the circular tents back in the boy scouts or in the army, where every man had their head facing outwards to the cold air and their feet facing the wood-burning stove in the center. But what if you wanted neat rows of bodybuilders, who didn’t curve into spheres? Well, you would align them facing the opposite directions, with the bodybuilder smelling the stinky feet of his neighbors, we would solve the problem of curving, even if we had to worry about stinky feet. The same can be thought of monolignols: the phenol groups are the broad shoulders, whereas the other side, called a propenol is relatively thin, like the legs. So, the phenol side is always facing the propenol side of the neighboring monolignol. But what about the tube-shape, isn’t that more like the soldiers in the tent, you ask? This is what tricked me for a while until I realized that what the phenol does is that it helps to carry the shopping bag of the propenol of the neighboring monolignol. You see, the hydrogen shopping bags are a curious bunch, as they tend to fall off. The next player in this game is the alcohol of the propenol. But it’s not alcohol, as a non-chemist perceives it, but a very similar thing to phenol, with oxygen holding hand with carbon and also holding the hydrogen shopping bag. In this case, the shopping bag is more tightly in the grips of the oxygen than with phenol, who likes to drop the bag every so often. But after the phenol has dropped his shopping bag, he has a free hand to help with the shopping bag of the propenol. This helping hand is called a hydrogen bond. Because of the hydrogen bond, the arrangement of the monolignols opposite each other becomes likely, or even inevitable.
But why the tube-shape, then? If the hydrogen bonding allows for the monolignols to take a linear shape, then isn’t it contradictory that you suddenly have a tube? This is something I couldn’t explain at first, but I nevertheless made a thought experiment of what would happen if such a tube formed. The feet of the bodybuilders facing outside would be inside the mouths of the bodybuilders facing inside, whereas there would be plenty of space on the outside for the feet to wiggle. In this compressed state, the only possible bond would be foot-in-mouth, or β-O-4, whereas on the outside, the knee of the bodybuilder is angled in a way that it’s almost possible to put the outer foot into the outer mouth.
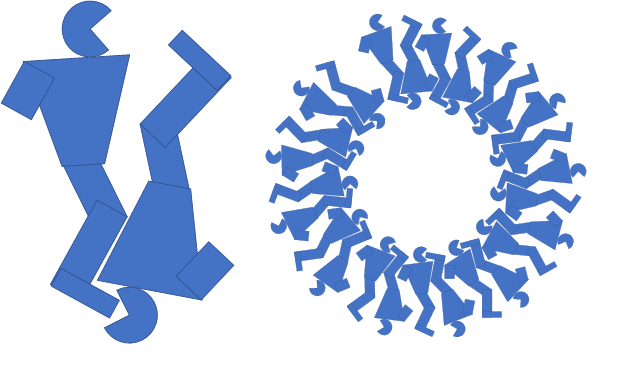
Foot-eating bodybuilders
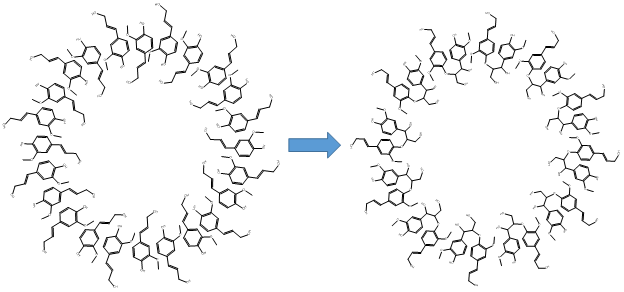
Actual β-O-4 bond
Or in a slightly more technical terms, the phenol groups would be pressed against the propenol in a way, that the most likely reaction to take place, if the double bond opened is for the β carbon to hold hand with the oxygen of the phenol. Conversely, on the stretched state, there would be no possibility for this kind of a β-O-4 bond. This was gold! In all of my scouring of the lignin literature, there was really no explanation for the prevalence of the β-O-4 bond, even though it was the most significant known feature of lignin. This meant that I was onto something.
But how could such a tube form? And how long was it? I really didn’t have a proper explanation for any of this. As it happened, I was thinking of these thoughts around the 1st of May, or May Day. Like in much of Europe, this day was a day of festivity. In Finland one of the treats prepared for the day are donuts. Having eaten a few donuts during the day, I was putting my boys to bed. My youngest one was two and finding it impossible to fall asleep by himself. Like on many another night, I was sitting next to his bed with nothing to do but to think. My mind was twisting the lignin tubes, first imagining them to be like nanoscale macaroni. But this didn’t make sense. The ends of the tubes could not be in the watery environment of the plant cell. Next, I thought of an extra-long hollow pasta, like bucatini. But still, there is always the problem of the end of the tube, which couldn’t be open. How about a closed bucatini? That would make some sense. But how long would such a bucatini be; would it be long or short? Then it hit me: not pasta, but a donut! But not any kind of a donut, a hollow one! What if the structure of lignin is first a tiny ring that only later opens up to form hollow tubes? All of these donuts would be of equal size and then fuse with each other into lattice. And not just any kind of a lattice, but a hexagonal one, because of close packing of the rings. I went to see whether anything like this had been published and to my amazement this is exactly the case. Instead, the people doing the research had no idea that lignin was responsible for the formation of such a lattice. The structure even had a name: hemicellulose lignin module, or HLM.
What else I found out about the structure of lignin, I'll have to leave to future posts.
Commentaires