I’ll begin by owning up that I had written a whole post before this, but as the point where I ended up in was so different to where I started from, I decided to just rewrite the whole post.
The biggest reason to rewrite the post is because my premise in the last post was wrong. First of all, the biggest problem is that I thought that the energy of the binding of the hydrogen molecules would be the 0.6 meV of the pairwise van der Waals bonding between hydrogen molecules. This was a reasonable first assumption, with just the flaw that it’s just wrong. But how do I know it?
I finally realized what the Rydberg formula means. In yesterday’s post I still didn’t, but I was already confident that I would. Today the pieces finally came together. Before explaining what the formula is all about, I’ll make a short detour to the nature of mathematics. Any equation with a minus sign is more or less an ‘accounting trick’ there are no negative physical entities. There are just elementary units of energy, or dots. So, if an equation has a minus sign, it means that a term has been removed from its ‘true’ place and located into a place where it is of use. But it hasn’t magically become negative. There are no negative dots.
In the Rydberg formula, this means that this:

is a ‘truer’ equation. It might seem that I’m being pedantic and splitting hairs, but this representation is actually crucial to understand what’s really going on. What the equation means in the context of absorption is that a supramolecular shell with a diameter of λvac/n’ absorbs a supraphoton with a diameter of λvac and releasing a huge number of hydrogen molecules from the initial shell, leaving behind a smaller shell, with a diameter of λvac/n. To give an example, if n’ is 1 (Lyman series) and n = ∞, we get:

This represents two distinct physical forms of energy, or strings of dots. The first energy, or πλvac is some what ‘truer’ measure of energy, as it represents the circumference of ring with a diameter of λvac. So, it could be called a low-abstraction energy: what you see is what you get (WYSIWYG).
The second term,

is more abstract. First of all it implies that there is an area of

This is an actual area. But it’s not correlated to energy in as tangible a way as that of the string. Rather, it requires a multiplier of RH, or the Rydberg constant to correlate the energy associated with the area with the more concrete energy of the ring of light.
And why is the area associated with a specific energy? Because each hydrogen molecule takes up a specific amount of area in the supramolecular shell, which means that the area depicts a specific number of molecules in the shell. Multiplication by RH allows us to have the energy required to break a single intermolecular bond between the hydrogen molecules times the number of these bonds.
And how do we draw this event? When n’ = 1 the ring of light (left, empty) is the same diameter as the supramolecular shell (middle, yellow). And on the right side of the arrow, you sort of have ‘nothing’, as the equation does not take into account the hydrogen molecules that have been released from the supramolecular shell. But not to confuse you, I drew a cloud of dots. These dots don’t represent anything numerical. I could have added any number of dots. The most important thing to note is that the number of hydrogen molecules hasn’t disappeared. However, the intrinsic energy of the molecules isn’t visible in the equation.
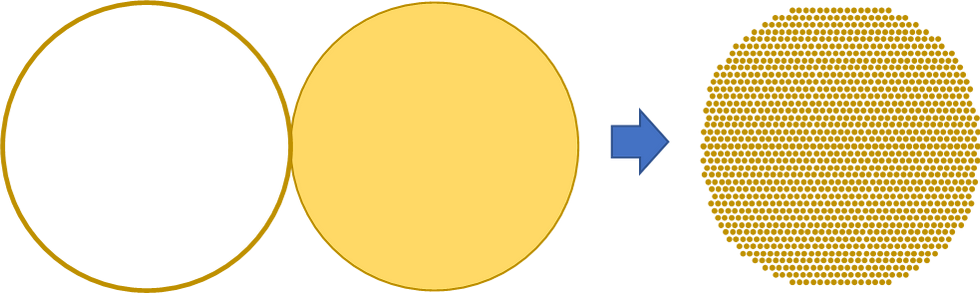
The next, slightly silly, question is why are the ring and the sphere both on the left side of the arrow, if the Rydberg equation puts the ring of light and the energy of the bonds on the right side? The well-known, but not necessarily intuitive, fact is that binding energy isn’t the presence of energy in the bond, but an absence of energy. Thus, to form a bond, you release energy. Using the terminology of a chemist, bonding is an exothermic process.
So is this process a chemical bond? Let’s look at the Wikipedia definition:
A chemical bond is a lasting attraction between atoms or ions that enables the formation of molecules, crystals, and other structures.
According to the above definition, this is definitely a chemical bond. So, what is the energy of this bond? It is 13.6 eV, or about 1312 kJ/mol. How does this relate to other chemical bonds? The H-H bond in a hydrogen molecule has a binding energy of 436 kJ/mol. Wait, what? The H-H bond, possibly the strongest covalent bond is one third in strength to the intermolecular bond! The intermolecular bond is stronger than the strongest covalent bond! Surely, this can’t be right?
Except that logic and the mathematics say this is exactly the case. But how is this even possible? The trick is that while the above scheme is mathematically true, the above scheme is mostly theoretical. The energy required for this transition is so high that it’s extremely difficult even to produce.
An interesting but also a bit sad case relating to this is the case of the hydrino. I might have talked about this before. A fellow by the name of Randell L. Mills observed that using extremely low wavelengths, he observed
high-energy light emission from hydrogen forming Hydrinos using a high-voltage pinched pulsed plasma source
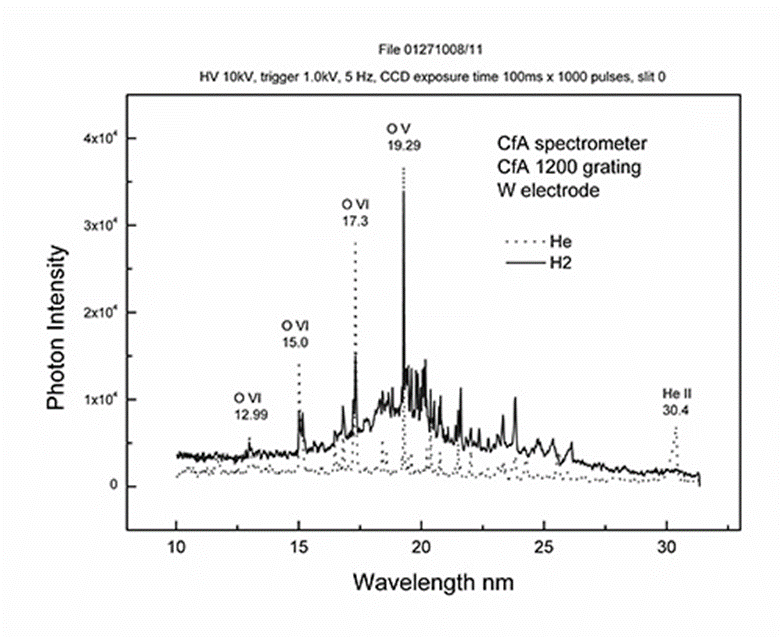
Mills was sure that what he had observed was dark matter. The scientific community accepted that he had indeed observed something, but his interpretation of his findings faced a lot of criticism.
I can’t say for sure, but it sure seems that Randell Mills found something real. The problem he had was that he didn’t have the mathematical tools to explain his findings and he ended up being ridiculed for his discoveries. That’s the problem with experimental science. If an experiment is difficult and you don’t know exactly what’s going on, it’s hard to convince others of your interpretations of these results.
But I was sidetracked. Even in the case of the hydrino, what was observed was emission. You can observe the absorption relating to the 1→2 transition (121.6 nm) from quasars, but not in from anything you could make in a lab. I’m not sure whether there has ever been observed the absorption relating to the 1→∞ transition (91.17 nm).
So how do we know of the 1→∞ transition line? We can have high vacuum with just hydrogen gas and high voltage current to ignite the gas. What the vacuum and the current do is allow hydrogen molecules to first be released from the supramolecular shell and then reform. The energy released in the formation of the supramolecular shell is released as a supraphoton with a wavelength of 91.17 nm.
But in real life, it’s much easier to produce emission bands from other transitions. For instance, the emission spectrum of a deuterium arc lamp (almost the same as a hydrogen lamp) looks like this.
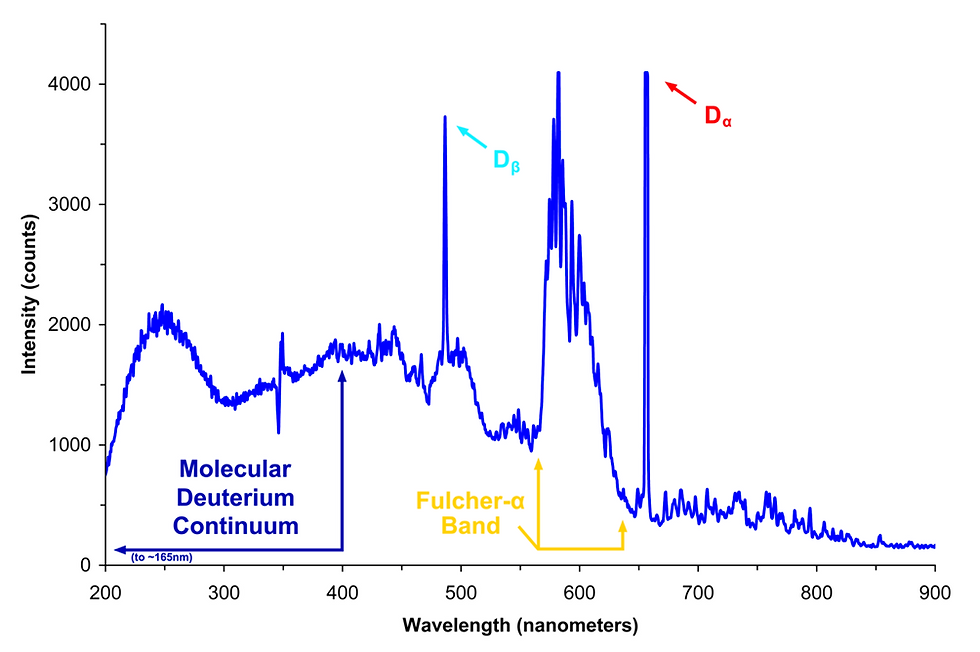
What you don’t see are just the bands relating to the relatively small number of transitions of the Balmer series. Rather, what you see is are the Balmer peaks, but also a broad continuous region of emission from ~160 nm to 400 nm and Fulcher band emission between 560 to 640 nm. Trying to explain this complex emission via just the Rydberg formula isn’t really possible, so I won’t even try.
So, is this a proper mathematical explanation of the Rydberg formula? I’d say, it’s a good start. In the original post I had quite a bit more of mathematical analysis on whether the model matches experimental data and I found that yes, it does. However, after explaining the mechanism this way, dumping a bunch of mathematics on you, dear reader, seems counterproductive. I think base on what I’ve discovered today, I’ll try to rewrite the Counterevidence paper and based on where I get stuck, I’ll write my next post.
But before signing out, I feel compelled to give a proper name to the phenomenon that up to now has been called energy level. Except, the energy level and the intermolecular bond aren’t really the same thing. The intermolecular bond (at least in hydrogen) always has a bonding energy of 13.6 eV (or 1312 kJ/mol). It’s just that depending on the specific conditions, not all of the bonds are broken. I was tempted to call the bond an orbital bond, but there is already a term called orbital bond index, that has been introduced for quantifying covalent bonding in solid-state materials. I was also considering calling it a shell bend, but there is already a concept called valence shell bonding, which relates to the outer shell of a the atom model, associated with chemical bonds. Perhaps a compromise could be an inner shell bond. To be frank, I’m not yet very keen on the term. Perhaps I’ll come up with a better name. Probably I’ll come up with a better name when I have a better understanding of what’s going on and how all of this relates to current theory.
And still one more thing. The curious property of the inner shell bond is that it always instantly reforms. So, there’s never a case more than a fraction of a second long, where molecules haven’t formed a shell bond with surrounding molecules. This means that the inner shell bond is the water that the fish are not aware of, as they have always swum in it.
Once again, I'm pretty excited about today's discovery. In some sense the difference between what I thought yesterday and today isn't that big, but on a deeper level, this realization is extremely profound. Still yesterday I thought that it was the weak 0.6 meV van der Waals bond keeping the supramolecular shell together, but today I understand that the bond is actually the strongest possible bond. So in a span of a single day I went from one extreme to another. I probably shouldn't say this but I say it anyhow: Today is a momentous day!
Comentarios