In today’s post I explore why my photovoltaic past didn’t work too well, or the distinction between an electron and a ring of photons.
During the past weeks I’ve become convinced that an electron is not the total orbiting mass of particles around the core of the proton/quarks, but only the length of string that can be released from the orbital. And this length of string isn’t just released from anywhere, but from the point of connection of two orbitals.
I’ll use an illustrated thought experiment to show what I mean. Below you see three identical orbitals touching. Conventionally one would consider what was orbiting to be an electron. However, if most of the mass of the proton isn’t in the quarks at the center, but in the ethereal ‘energy’, then the energy must be the fraction of orbital that is never released. In this illustration you cannot see an electron, as nothing has been detached from either of the orbital.
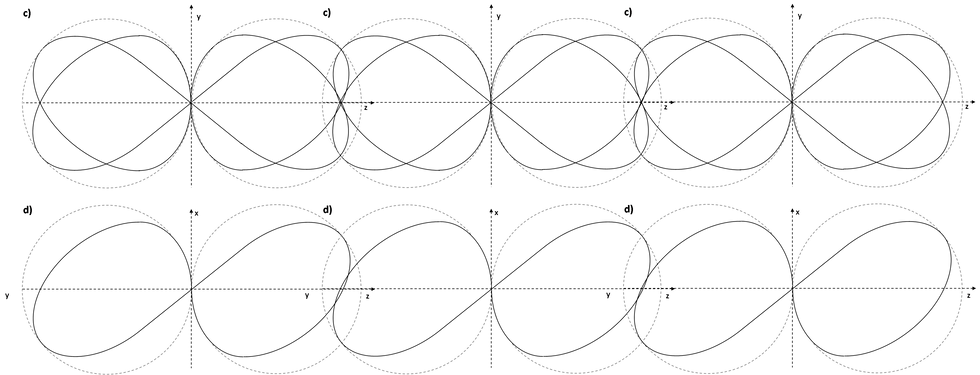
In my previous posts I’ve proposed that the supramolecular orbital to be a closed loop of interconnected orbitals. These orbitals are connected at the point where two loops intersect. So, what happens if you twist one of these orbitals around? You form a tiny orbital around both sides of the twisted orbital, a bit like twisting balloon animals.
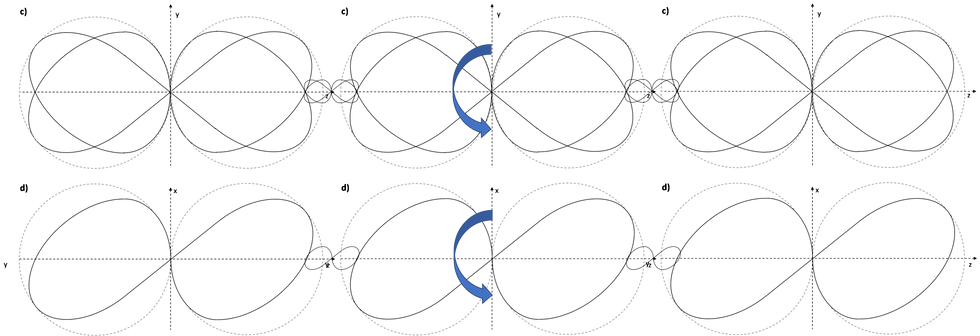
Now, it’s important to understand that not all orbitals give out electrons, at least not very easily. Whether orbits twist to form electrons or not depends on a lot of factors. The mathematics and experimental basis of all of this has been studied extensively since the days of Alessandro Volta, so I won’t bore you too much about that.
Chromophores are molecules that can capture the energy of light and convert it to mass. And not only mass: if the conditions are correct, they release the mass back as electrons. Next, I’ll explore how this is done.
Using chlorophyll as an example again, I’ll try to think how a band of light is captured by the rod of chlorophyll and what happens to the electrons generated. As far as I currently understand it, the ring of blue light conforms to the shape of the rod of chlorophyll in chloroplast and begins spinning the chlorophyll molecules in the rod, a bit like they spin rows of prayer wheels in Nepal. This spinning is not just the turning of the chlorophyll molecule in place, as it won’t turn if not given ‘loose string’ to be allowed to turn. Thus, the ring of light that is absorbed by the rod is turned into electrons by the process of incorporating the string as fragments into the orbital.
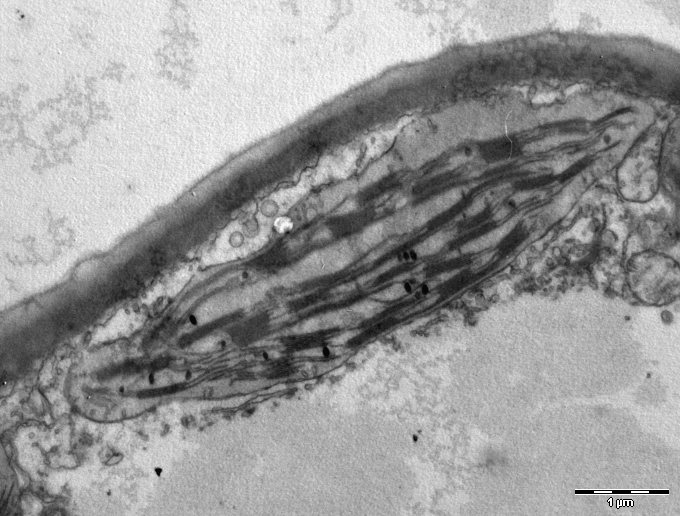
But here it’s crucial to understand that the chlorophyll molecules in the rod aren’t independent, but part of a single supramolecular shell, with the interactions moving from one molecule to another at the speed of light. So, it isn’t just one molecule spinning, but the spinning of the outer molecules affects the spinning of all of the molecules in the rod.
So, one piece of the ring of light absorbed by a single molecule of chlorophyll is a photon. But the number of photons absorbed by the rod is not the same as the number of electrons generated. Or more specifically, an electron is always the same size, but the number of electrons released depends on the size of the ring of light.
Ok, now we have the surface of the rod of chlorophyll covered in electrons. Then what? First of all, you have photosystem II. This means that while chlorophyll is present as supramolecular shells, they are not isolated from other parts required for photosynthesis. Honestly, I haven’t studied the structure of this system to a sufficient degree to say much more. Suffice it to say that to get the electrons out requires enzymes to capture the energy of the absorbed ring of light.
In photochemistry, there is a difference between energy transfer and electron transfer. In practice, this means that if there is energy transfer, the twisted chlorophylls don’t generate a sufficiently long loop to make an electron, but these loops need to be collected by an enzyme to be combined into a sufficiently long loop to make up an electron.
Enzymes aren’t the only option for energy transfer for all photosystems. In man-made systems you can replace enzymes with synthetic molecules, such as fullerene. This is what I did when building organic solar cells.
So, chlorophyll captures a ring of light and the part of the ring is transferred to enzymes that converts the light to an electron. After this you have a long way to go until you get sugar. For instance you have a second absorption of light, which is confusingly called photosystem I, which generates ATP. You might possibly remember from high school biology class, that this is what powers everything in cells. In the final stage you have the Calvin cycle, or a ‘dark reaction’, which generates sugars from water and carbon dioxide. I won’t try to go deeper than that.
So, what is a proton? It’s a hydrogen atom unscrewed from the supramolecular shell, at least when ‘free’. This means that in most cases you can’t remove a whole hydrogen atom without leaving an electron ‘stub’ in the supramolecular orbital. However, it is possible to generate single hydrogen atoms. These are called hydrogen radicals. But they are highly reactive and much less common than protons, which can be found just about everywhere and play an important role both in life and in synthetic chemistry.
Then there is the question of electrons and electricity. They’re the same, right? What we usually think of electricity is electric current, which is much more like light than electrons. You, see electrical current flows, whereas electrons react. This is a bit of a simplification, but not too far off. You can have electrical current in an electrical conductor. These are mostly specific metals. Semiconductors, on the other hand can conduct a bit of current, but not well enough to make power cables out of. Or more specifically semiconductors have many other nice properties that make them useful, but not as wires.
I was about to write about my idea of what the physical characteristic of voltage is but ended up rather insecure about it. If current is the net rate of flow through the electrical wire, voltage has to be the density of Planck sphere in the wire. If the ring is extra-large, there can be only a small number of rings in a cross-sectional area and thus a small density of Planck sphere per area. Conversely, if the rings are very small, there can be a large number of rings in a cross-sectional area. This seems to imply that that a high voltage translates to small rings and low voltage translates to large rings.
But I don’t even need to use a thought experiment. I can just go to the literature and see the standard calculations that back this interpretation up. For instance, the calculation of the minimum voltage required to produce and x-ray with a wavelength of 0.38 nm can be calculated with the equation

, where q is the charge of an electron and V the voltage by which it is accelerated. Ignoring the calculations for no, we get a minimum voltage of 32710.5 V. All this is just standard knowledge
What I claim that if you pass a current of 32710.5 V in an power cable (it has to be quite sturdy), what is moving in the cable is an x-ray of 0.38 nm. Even this isn’t exactly new information, but even the Wikipedia page is a bit confusing. What this means is that the physics is surely correct, but the ‘story’ is told in a confusing way. As far as I understand it, the general knowledge is that the rings of ‘light’ (or ionizing radiation, when talking about power cable level voltages) move electrons (and holes) in the cable.
So, combining present knowledge with the of the ring and orbital -model of electricity, current becomes the hitting of electrons with circular ‘bats of light’. Even though I spent five years of my life studying a small part of this process, I have to say my understanding at the time was quite sketchy. The problem is that if you have to rely on mathematics to make things understandable, you don’t form a clear picture in you mind what you’re actually doing. This means that while you’re technically right, you can’t change the system in the optimal way, because you really don’t know what you’re doing.
Nowadays the research in organic solar cells has taken a back seat and my colleagues who remained studying solar cells, switched to perovskite solar cells, which is hybrid organic-inorganic structure. This is to say that despite thousands of publications of organic solar cells, it was finally realized that there are better ways of doing things. This goes to show how little we know and how chemistry is still very much an educated system of trial-and-error.
Comments